Figure 7y-1 illustrates the
basic components that influence the state of the
Earth's climatic system. Changes in the state of
this system can occur externally (from extraterrestrial
systems) or internally (from ocean, atmosphere and
land systems) through any one of the described components.
For example, an external change may involve a variation
in the Sun's output which would externally vary the
amount of solar
radiation received by the Earth's atmosphere
and surface. Internal variations in the Earth's climatic
system may be caused by changes in the concentrations
of atmospheric gases, mountain building, volcanic
activity, and changes in surface or atmospheric albedo.
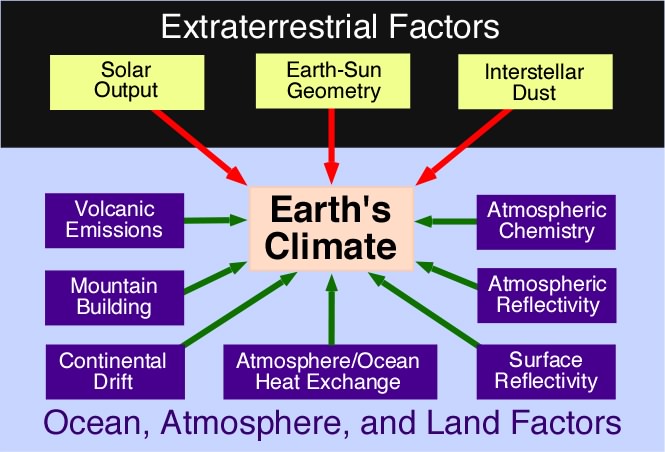
Figure
7y-1: Factors
that influence the Earth's climate.
The work of climatologists has found
evidence to suggest that only a limited number of
factors are primarily responsible for most of the
past episodes of climate change on the Earth. These
factors include:
- Variations in the Earth's orbital characteristics.
- Atmospheric carbon dioxide variations.
- Volcanic eruptions
- Variations in solar output.
Variations in the Earth's Orbital
Characteristics
The Milankovitch theory suggests
that normal cyclical variations in three of the
Earth's orbital characteristics is probably responsible
for some past climatic change. The basic idea
behind this theory assumes that over time these
three cyclic
events vary the amount of solar
radiation that is received on the Earth's
surface.
The first cyclical variation, known
as eccentricity,
controls the shape of the Earth's orbit around the
Sun. The orbit gradually changes from being elliptical
to being nearly circular and then back to elliptical
in a period of about 100,000 years. The greater the
eccentricity of the orbit (i.e., the more elliptical
it is), the greater the variation in solar energy
received at the top of the atmosphere between the
Earth's closest (perihelion)
and farthest (aphelion)
approach to the Sun. Currently, the Earth is experiencing
a period of low eccentricity. The difference in the
Earth's distance from the Sun between perihelion
and aphelion (which is only about 3%) is responsible
for approximately a 7% variation in the amount of
solar energy received at the top of the atmosphere.
When the difference in this distance is at its maximum
(9%), the difference in solar energy received is
about 20%.
The second cyclical
variation results from the fact that, as the Earth
rotates on its polar
axis, it wobbles like a spinning top changing
the orbital timing of the equinoxes and solstices (see Figure 7y-2 below).
This effect is known as the precession
of the equinox. The precession
of the equinox has a cycle of approximately
26,000 years. According to illustration A,
the Earth is closer to the Sun in January (perihelion) and farther away
in July (aphelion)
at the present time. Because of precession, the
reverse will be true in 13,000 years and the Earth
will then be closer to the Sun in July (illustration
B).
This means, of course, that if everything else
remains constant, 13,000 years from now seasonal
variations in the Northern Hemisphere should be
greater than at present (colder winters and warmer
summers) because of the closer proximity of the
Earth to the Sun.

Figure
7y-2: Modification
of the timing of aphelion and perihelion
over time (A = today; B =
13,000 years into the future). |
The third cyclical variation is related
to the changes in the tilt (obliquity) of the Earth's axis
of rotation over a 41,000 year period. During the
41,000 year cycle the tilt can deviate from approximately
22.5 to 24.5°. At the present time, the tilt
of the Earth's axis is 23.5°. When the tilt
is small there is less climatic variation between
the summer and winter seasons in the middle and
high latitudes. Winters tend to be milder and summers
cooler. Warmer winters allow for more snow to
fall in the high latitude regions. When the atmosphere
is warmer it has a greater ability to hold water
vapor and therefore more snow is produced at areas
of frontal or orographic
uplift. Cooler summers cause snow and ice
to accumulate on the Earth's surface because less
of this frozen water is melted. Thus, the net effect
of a smaller tilt would be more extensive formation
of glaciers in the polar latitudes.
Periods of a larger tilt result in
greater seasonal climatic variation in the middle
and high latitudes. At these times, winters tend
to be colder and summers warmer. Colder winters produce
less snow because of lower atmospheric temperatures.
As a result, less snow and ice accumulates on the
ground surface. Moreover, the warmer summers produced
by the larger tilt provide additional energy to melt
and evaporate the snow that fell and accumulated
during the winter months. In conclusion, glaciers
in the polar regions should be generally receding,
with other contributing factors constant, during
this part of the obliquity cycle.
Computer models and historical evidence
suggest that the Milankovitch cycles exert their
greatest cooling and warming influence when the troughs
and peaks of all three cycles coincide with each
other.
Atmospheric Carbon Dioxide Variations
Studies of long term climate change
have discovered a connection between the concentration
of carbon
dioxide in the atmosphere and mean global
temperature. Carbon dioxide is one of the more important
gases responsible for the greenhouse
effect. Certain atmospheric gases, like carbon
dioxide, water vapor and methane,
are able to alter the energy balance of the Earth
by being able to absorb longwave radiation emitted
from the Earth's surface. The net result of this
process and the re-emission of longwave back to
the Earth's surface increases the quantity of heat
energy in the Earth's climatic system. Without
the greenhouse effect, the average global temperature
of the Earth would be a cold -18° Celsius rather than the
present 15° Celsius.
Researchers of the 1970s CLIMAP
project found strong evidence in deep-ocean
sediments of variations in the Earth's global
temperature during the past several hundred thousand
years of the Earth's history. Other subsequent
studies have confirmed these findings and have
discovered that these temperature variations
were closely correlated to the concentration
of carbon dioxide in the atmosphere and variations
in solar radiation received by the planet as
controlled by the Milankovitch cycles. Measurements
indicated that atmospheric carbon dioxide levels
were about 30% lower during colder glacial periods.
It was also theorized that the oceans were a
major store of carbon dioxide and that they controlled
the movement of this gas to and from the atmosphere.
The amount of carbon dioxide that can be held
in oceans is a function of temperature. Carbon
dioxide is released from the oceans when global
temperatures become warmer and diffuses into
the ocean when temperatures are cooler. Initial
changes in global temperature were triggered
by changes in received solar radiation by the
Earth through the Milankovitch cycles. The increase
in carbon dioxide then amplified the global warming
by enhancing the greenhouse effect.
Over the past three
centuries, the concentration of carbon dioxide
has been increasing in the Earth's atmosphere because
of human influences (Figure 7y-3). Human
activities like the burning of fossil fuels, conversion
of natural prairie to farmland, and deforestation
have caused the release of carbon dioxide into
the atmosphere. From the early 1700s, carbon dioxide
has increased from 280 parts per million to 380
parts per million in 2005. Many scientists believe
that higher concentrations of carbon dioxide in
the atmosphere will enhance the greenhouse effect
making the planet warmer. Scientists believe we
are already experiencing global warming due to
an enhancement of the greenhouse effect. Most computer
climate models suggest that the globe will warm
up by 1.5 - 4.5° Celsius
if carbon dioxide reaches the predicted level of
600 parts per million by the year 2050.
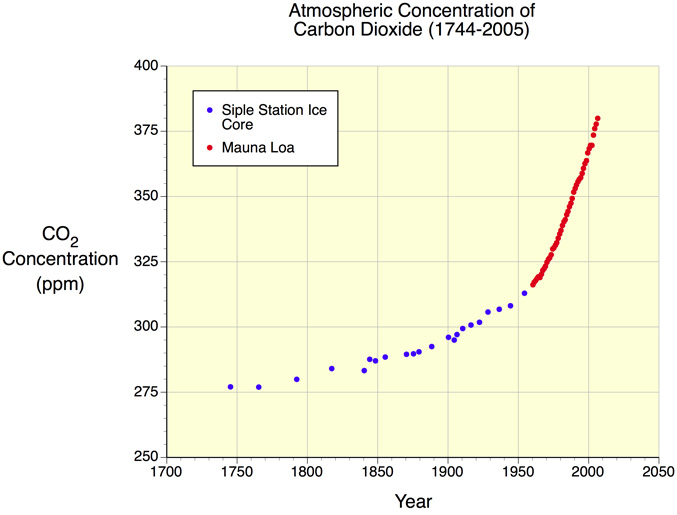
Figure
7y-3: The
following graph illustrates the rise in
atmospheric carbon dioxide from 1744
to 2005. Note that the increase in
carbon dioxide's concentration in the
atmosphere has been exponential during
the period examined. An extrapolation
into the immediate future would suggest
continued increases.
(Source: Neftel,
A., H. Friedli, E. Moore, H. Lotscher,
H. Oeschger, U. Siegenthaler, and B.
Stauffer. 1994. Historical carbon dioxide
record from the Siple Station ice core.
pp. 11-14. In T.A. Boden, D.P. Kaiser,
R.J. Sepanski, and F.W. Stoss (eds.) Trends'93:
A Compendium of Data on Global Change.
ORNL/CDIAC-65. Carbon Dioxide Information
Analysis Center, Oak Ridge National Laboratory,
Oak Ridge, Tenn. U.S.A. and
Keeling,
C.D. and T.P. Whorf. 2006. Atmospheric
CO2 records from
sites in the SIO air sampling network.
In Trends: A Compendium of Data
on Global Change. Carbon Dioxide
Information Analysis Center, Oak Ridge
National Laboratory, U.S. Department
of Energy, Oak Ridge, Tenn., U.S.A.). |
Volcanic Eruptions
For many years, climatologists have
noticed a connection between large explosive volcanic eruptions
and short term climatic change (Figure 7y-4).
For example, one of the coldest years in the last
two centuries occurred the year following the Tambora
volcanic eruption in 1815. Accounts of very cold
weather were documented in the year following this
eruption in a number of regions across the planet.
Several other major volcanic events also show a pattern
of cooler global temperatures lasting 1 to 3 years
after their eruption.
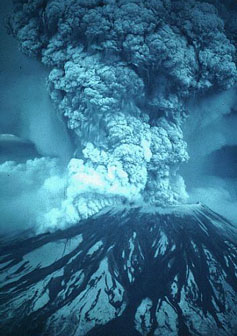
Figure
7y-4: Explosive
volcanic eruptions have been shown to have
a short-term cooling effect on the atmosphere
if they eject large quantities of sulfur dioxide
into the stratosphere. This image shows the
eruption of Mount St. Helens on May 18, 1980
which had a local effect on climate because
of ash reducing the reception of solar radiation
on the Earth's surface. Mount St. Helens had
very minimal global effect on the climate because
the eruption occurred at an oblique angle putting
little sulfur dioxide into the stratosphere.
(Source: U.S.
Geological Survey, photograph by Austin Post). |
At first, scientists thought that the
dust emitted into the atmosphere from large volcanic
eruptions was responsible for the cooling by partially
blocking the transmission of solar
radiation to the Earth's surface. However,
measurements indicate that most of the dust thrown
in the atmosphere returned to the Earth's surface
within six months. Recent stratospheric data
suggests that large explosive volcanic eruptions
also eject large quantities of sulfur
dioxide gas which remains in the atmosphere
for as long as three years. Atmospheric chemists
have determined that the ejected sulfur dioxide gas
reacts with water vapor commonly found in the stratosphere
to form a dense optically bright haze layer that
reduces the atmospheric transmission of some of the
Sun's incoming radiation.
In the last century, two significant
climate modifying eruptions have occurred. El Chichon
in Mexico erupted in April of 1982, and Mount Pinatubo
went off in the Philippines during June, 1991 (Figure
7y-5). Of these two volcanic events, Mount Pinatubo
had a greater effect on the Earth's climate and ejected
about 20 million tons of sulfur dioxide into the
stratosphere (Figure 7y-6). Researchers believe
that the Pinatubo eruption was primarily responsible
for the 0.8 degree Celsius drop in global average
air temperature in 1992. The global climatic effects
of the eruption of Mount Pinatubo are believed to
have peaked in late 1993. Satellite data confirmed
the connection between the Mount Pinatubo eruption
and the global temperature decrease in 1992 and 1993.
The satellite data indicated that the sulfur dioxide
plume from the eruption caused a several percent
increase in the amount of sunlight reflected by the
Earth's atmosphere back to space causing the surface
of the planet to cool.
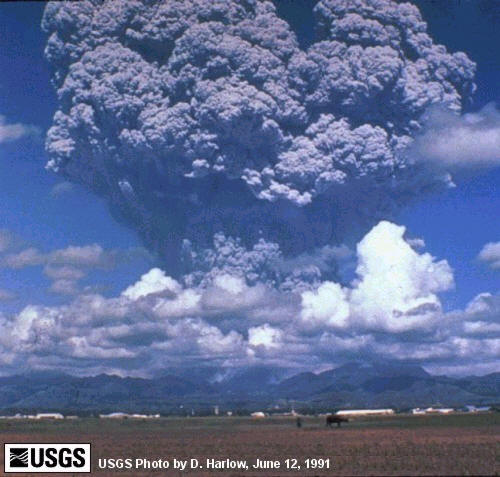
Figure
7y-5: Ash
column generated by the eruption of Mount Pinatubo
on June 12, 1991. The strongest eruption of
Mount Pinatubo occurred three days later on
June 15, 1991. (Source: US
Geological Survey). |
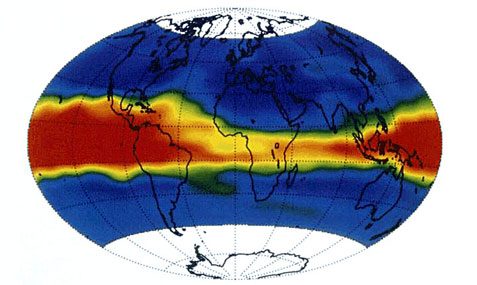
Figure
7y-6: The
following satellite image shows the distribution
of Mount Pinatubo's sulfur dioxide and dust
aerosol plume (red and yellow areas) between
June 14 and July 26, 1991. Approximately
45 days after the eruption, the aerosol
plume completely circled the Earth around
the equator forming a band 20 to 50° of
latitude wide. Areas outside this band
were clear of volcanic aerosols. Within
a year, the sulfur dioxide continued to
migrate towards the North and South Pole
until it covered the entire Earth because
of the dominant poleward flow of stratospheric
winds (stratospheric winds circulate from
the equator to the polar vortices at the
North and South Poles). These observed
patterns of aerosol movement suggest that
tropical explosive volcanic eruptions probably
have the greatest effect on the Earth's
climate. Diffusion of aerosols by stratospheric
winds from a tropical source results in
the greatest latitudinal coverage of the
sulfur dioxide across both the Northern
and Southern Hemispheres. (Source: SAGE
II Satellite Project - NASA). |
Variations in Solar Output
Until recently, many scientists thought
that the Sun's output of radiation only varied by
a fraction of a percent over many years. However,
measurements made by satellites equipped with radiometers in
the 1980s and 1990s suggested that the Sun's
energy output may be more variable than was once
thought (Figure 7y-7). Measurements made
during the early 1980s showed a decrease of 0.1
percent in the total amount of solar energy reaching
the Earth over just an 18 month time period. If
this trend were to extend over several decades,
it could influence global climate. Numerical climatic
models predict that a change in solar output of
only 1 percent per century would alter the Earth's
average temperature by between 0.5 to 1.0° Celsius.
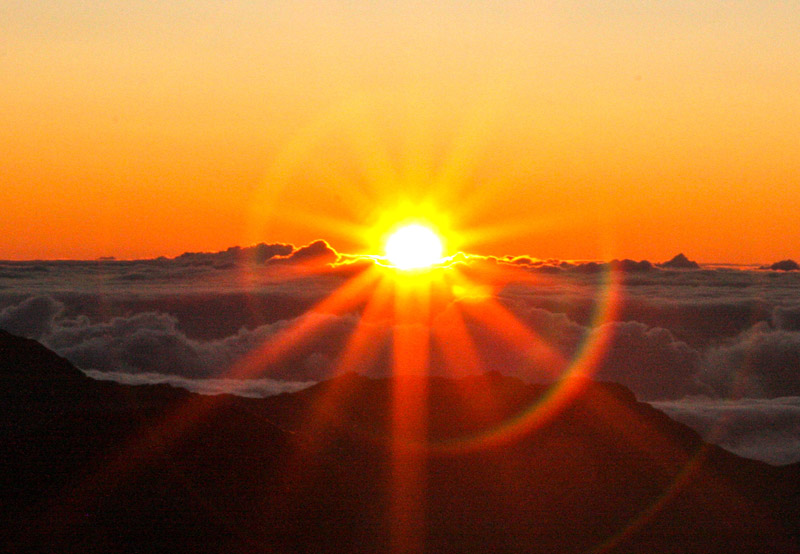
Figure
7y-7: The
Sun as seen at sunrise. The Sun is essentially
the only source of energy for running the Earth's
climate. Thus any change in its output will
result in changes in the reception of insolation
and the generation of heat energy which drives
the climate system. |
Scientists have long tried to also
link sunspots to
climatic change (also see the link www.sunspotcycle.com).
Sunspots are huge magnetic storms that are seen
as dark (cooler) areas on the Sun's surface. The
number and size of sunspots show cyclical patterns,
reaching a maximum about every 11, 90, and 180
years. The decrease in solar energy observed in
the early 1980s correspond to a period of maximum
sunspot activity based on the 11 year cycle. In
addition, measurements made with a solar telescope
from 1976 to 1980 showed that during this period,
as the number and size of sunspots increased, the
Sun's surface cooled by about 6° Celsius. Apparently,
the sunspots prevented some of the Sun's energy
from leaving its surface. However, these findings
tend to contradict observations made on longer
times scales. Observations of the Sun during the
middle of the Little Ice Age (1650 to 1750) indicated
that very little sunspot activity was occurring on
the Sun's surface. The Little
Ice Age was a time of a much cooler global
climate and some scientists correlate this occurrence
with a reduction in solar activity over a period
of 90 or 180 years. Measurements have shown that
these 90 and 180 year cycles influence the amplitude
of the 11 year sunspot cycle. It is hypothesized
that during times of low amplitude, like the Maunder
Minimum, the Sun's output of radiation is
reduced. Observations by astronomers during this
period (1645 to 1715) noticed very little sunspot
activity occurring on the Sun.
During periods of maximum sunspot activity,
the Sun's magnetic field is strong. When sunspot
activity is low, the Sun's magnetic field weakens.
The magnetic field of the Sun also reverses every
22 years, during a sunspot minimum. Some scientists
believe that the periodic droughts on the Great Plains
of the United States are in someway correlated with
this 22 year cycle.