Summary of the
Chapter
This chapter introduces the student to
the study of climatology and meteorology. The chapter
begins with an examination of the composition and structure
of the atmosphere. According to temperature change
with altitude, seven different layers can be identified
in the atmosphere. The lowest layer, the troposphere,
extends from the surface to a height of 11 kilometers.
This layer contains the majority of the atmosphere's
mass and is the location for most of the Earth's weather.
Characteristics of the other six layers are described
in detail.
The gases nitrogen and oxygen together
make up about 99% of the volume of the dry atmosphere.
The remaining 1% is comprised of a number different
gases of which ozone, carbon dioxide, nitrous oxide,
and methane are the most important to life on the Earth.
Ozone is concentrated in a layer that extends from
15 to 55 kilometers above the Earth's surface. Ozone
is important to life because it absorbs harmful ultraviolet
radiation from the Sun. Recent investigations of the
ozone layer have discovered areas of severe thinning
located primarily at the South Pole. Researchers have
determined that this thinning is caused by the emission
of the artificially produced chemical chlorofluorocarbon
into our atmosphere.
Several gases found in the atmosphere
have the ability to absorb infrared radiation from
the Earth's surface and atmosphere giving rise to the
greenhouse effect. The greenhouse effect aids in the
heating of the Earth's atmosphere and surface. Without
it, the average temperature of the planet would be
33 degrees Celsius colder. Three important gases involved
in the greenhouse effect process are methane, carbon
dioxide, and nitrous oxide. The concentration of these
gases in our atmosphere has been increasing since the
beginning of the Industrial Revolution primarily because
of the burning of fossil fuels. Other gases involved
in the greenhouse effect include: ozone, water vapor,
and chlorofluorocarbons.
A number of factors can influence the
intensity of the solar radiation received at the Earth's
surface. In the previous chapter (6.
Matter and Energy), we discovered that the
Sun can vary in its output of radiation and that a
variety of geometrical relationships between the Earth
and the Sun have considerable effect on the intensity
and duration of incoming solar radiation. As the solar
radiation passes through the Earth's atmosphere the
processes of scattering, absorption, and reflection
can also reduce the intensity of the shortwave beam.
The shortwave energy received by the
Earth is balanced by a similar quantity of longwave
radiation leaving back to space. This process is called
the planetary energy balance. In this chapter, models
of global shortwave and longwave dynamics were developed.
Latitudinally, amounts of incoming shortwave and outgoing
longwave radiation are not balanced. From 30 degrees
North to 30 degrees South incoming shortwave radiation
exceeds outgoing terrestrial radiation creating a surplus
of energy at these latitudes. At latitudes 30 to 90
degrees North and South the reverse holds true and
these regions have a deficit of energy. Several systems,
like oceanic and atmospheric circulation, act to redistribute
the surplus of energy at the tropics to the middle
and high latitudes.
Radiation energy absorbed at the Earth's
surface or within its atmosphere is normally converted
into a number of different forms of energy and used
in a variety of natural processes. One important conversion
involves the creation of heat energy that is used to
warm the Earth's surface and atmosphere. The generation
of heat energy is strongly correlated to the quantity
of shortwave radiation received. As discussed earlier,
the amount of insolation being received by a location
on the Earth varies both spatially and temporally because
of Sun-Earth geometry, Earth rotation, and spatial
differences in the Earth's atmospheric transparency.
Temperature can be defined as the intensity
or degree of hotness of a body. A number of measurement
scales have been invented to measure temperature. Heat
is a measure of the quantity of heat energy present
in a body. The heat contained in a body depends not
only on its temperature but also its mass. Daily and
annual fluctuations in temperature at the Earth's various
locations is caused by variations in the input and
output of net radiation. Spatial and temporal patterns
of temperature are also influenced by factors like
altitude, ocean currents, and surface properties.
Wind can be simply defined as air in
motion. Air moves above the Earth's surface because
of spatial differences in the density of the atmosphere.
Newton's laws of motion suggest that wind should blow
from areas of high density to areas of low density.
We can measure the density of the air through atmospheric
pressure. The speed of wind is controlled by pressure
gradient force. Pressure gradient force can be simply
described as the rate of pressure change (pressure
gradient) over space.
Once in motion air is influenced by a
number of forces. The rotation of the Earth causes
medium and large scale movements of air to be deflected
from their intended path. The magnitude of this force,
known as Coriolis force, is controlled by the latitude
of the location and the speed of the wind. Another
force that acts on wind is centripetal force. This
force is active when wind is flowing around curved
paths, and high or low pressure centers. The final
force that can influence moving air is friction. However,
friction only acts on wind that is flowing near the
surface of the Earth.
A variety of different types of large
scale winds have been described by scientists. A geostrophic
wind occurs in regions of the atmosphere (1 kilometer
above the Earth's surface) where friction is low and
the air tends to flow in a straight path. In geostrophic
winds, only two forces are active: pressure gradient
force and Coriolis force. Winds blowing in the upper
atmosphere in curved paths are called gradient winds.
These winds are influenced by the same forces as geostrophic
winds plus the effect of centripetal force. Winds near
the Earth's surface are called friction layer winds.
Friction layer winds are the result of pressure gradient
force, Coriolis force, centripetal force, and frictional
force.
There are a number of unique types of
circulation that exist at local and regional scales.
These winds are primary the result of thermally generated
circulation systems. In these systems, warm temperatures
create areas of low atmospheric pressure on the Earth's
surface, while areas of high pressure are generated
when temperatures are relatively cold. Once established,
the thermal gradient then develops a flow of air that
goes from high to low pressure on the Earth's surface.
Winds of this sort include, land and sea breeze, mountain
and valley breeze, and on a regional scale monsoon
winds.
At the global scale, consistent patterns
of air flow can be seen at the Earth's surface and
within its upper atmosphere. Global winds develop because
of latitudinal variations in atmospheric pressure.
However, these differences in pressure are not just
the result of the differential heating of the Earth's
surface. At approximately 30 degrees North and South
latitude, the subtropical high pressure zone forms
because of the presence of descending air from the
upper atmosphere. The sub-polar lows, located at about
60 degrees North and South latitude, develop because
of the dynamic interaction of cold polar air with warm
moist subtropical air masses. This interaction causes
frontal lifting and the development of cyclonic storms.
Surface winds move from areas of high pressure to low
pressure. The course of this movement is also altered
by the influence of Coriolis force causing the development
of the trade winds (0 to 30 degrees N and S), the westerlies
(30 to 60 degrees N and S) and the polar easterlies
(60 to 90 degrees N and S). Upper atmosphere winds
are generally poleward and westerly direction. Their
development is related to the presence of the Hadley,
Ferrel and Polar circulation cells in the North and
South hemisphere. Associated with the upper air winds
are narrow regions of intensification where fast moving
air is channeled into west to east flowing jet streams.
Jet streams can be found over the subtropical high
zones and the sub-polar lows.
An air mass is a large region of surface
air of similar temperature and humidity characteristics.
These masses of air move across the planet (as a part
of global circulation) influencing the regional climate
of regions of the globe for days to weeks. Climatologists
have classified air masses based on their air temperature
and moisture characteristics. The meeting of two air
masses frequently produce a front. At these frontal
zones differences in temperature can cause one mass
to be displaced over another. The four main types of
fronts are: stationary, cold, warm and occluded.
Mid-latitude cyclones are storms that
develop when cold polar air interacts with subtropical
air in the mid-latitudes. The result of this interaction
is the development of a large (2000 km in diameter)
rotating vortex of air with low atmospheric pressure.
Also, common with these storms is the development of
cold and warm fronts that are positioned to form a
wave. Precipitation is found at the center of the storm
and along the fronts where warm air is lifted over
cooler air.
Thunderstorms are small (1 to 10 kilometers
in diameter) storms that develop mainly in the tropics
and in the mid-latitudes. Thunderstorms form when moist,
unstable air is lifted vertically in to the atmosphere.
The lifting process causes the moist air to cool causing
condensation, cumulus cloud development and the release
of latent heat. If enough moisture is available, the
release of latent heat can enhance the vertical development
of these storms to the top of the troposphere producing
a cumulonimbus cloud. Development of the storm ends
normally in a few hours when most of the water vapor
is converted into precipitation. At this point, gravity
and internal circulation then directs the precipitation
to the ground surface producing heavy rain. Some mid-latitude
thunderstorms can be converted into severe storms through
additional uplift due to the presence of a mid-latitude
cyclone. Severe thunderstorms can have hail, strong
winds, thunder, lightning, intense rain, and tornadoes.
Weather in the tropics is characterized
by convective storms that develop with the intertropical
convergence zone, the hot clear weather associated
with the subtropical high pressure zone, and severe
oceanic cyclonic storms known as hurricanes. The animation
in section 7u illustrates
the seasonal movements of the intertropical convergence
zone and subtropical high pressure zone. Note that
areas near the equator tend to be under the influence
of the intertropical convergence longer than areas
further away. This phenomenon also causes regions away
from the equator to experience distinct wet and dry
seasons. Hurricanes are intense cyclonic storms that
only develop over the tropical oceans. Atmospheric
pressure at the center of these storms averages about
950 millibars. This extremely low pressure creates
intense damaging winds. Hurricanes are composed of
numerous bands of thunderstorms, which are produced
by the release of latent heat from the condensation
process. The lifespan of these storms is usually about
7 days. Most storms die when they are cutoff from their
supply of latent heat energy. This occurs with landfall
or when the storm moves to cooler ocean surfaces.
The Koppen climate classification system
is the most widely used system for classifying the
Earth's climatic types. This system recognizes five
major types of climate. From the equator to the poles
we have: A - Tropical Moist Climates; B - Dry Climates;
C - Mid-Latitude Climates with Mild Winters; D - Mid-Latitude
Climates with Cold Winters; and E - Polar Climates.
Each one of these climates has sub-classifications
that generally differ in the timing and quantity of
their precipitation.
A number of factors influence the climate
of a location. These factors include: a) Latitude and
its influence on solar radiation received; b) Air mass
influences; c) Location of high and low pressure systems;
d) Heat exchange from ocean currents; e) Location of
mountain barriers; f) Pattern of prevailing winds (including
monsoons); g) Distribution of land and sea; and h)
Altitude. The first three of these factors is examined
in relationship with the Koppen climate classification
system and the climatic data for some selected locations
on the Earth.
Urban climates differ from rural climates.
In general, urban climates tend to be warmer, have
more precipitation and different wind patterns. The
urban environment modifies its climate in a number
of ways: 1) the surface materials in cities absorb
more shortwave radiation; 2) configuration of building
increases the absorption of shortwave radiation at
low Sun angles and reduces the loss of longwave radiation
at night; 3) transportation, industry and the heat
of buildings artificially warm the air surrounding
cities; 4) precipitation is higher in cities because
increased thermal uplift and because of a greater quantity
of condensation nuclei (dust); and 5) winds are for
the most part reduced by the increased friction of
the city surface. However, some street/building configurations
can increase with speeds through tunneling.
Scientists are busy reconstructing past
climatic conditions because of the belief that the
past is the key to our future. There are three main
categories of data used in this reconstruction: 1)
meteorological records; 2) written documents and descriptive
accounts; and 3) physical and biological proxy data.
Meteorological records provide measurements of specific
climatic variables on a variety of temporal and spatial
scales. A standardized global network of weather stations
started developing at the turn of this century. This
network, however, has some problems due to short records
of observation and a lack of stations over the world's
oceans and in less developed countries. Recent advances
in instrumentation have allowed us to gather climatic
data globally from satellites. Written documents and
descriptive accounts of weather are normally very subjected.
However, some accounts describe features of climatic
change that are based on biological or physical phenomena.
These accounts are much more creditable. For example,
blossom dates of trees, dates of water body freezing,
and effects on crops. Scientists have used a variety
of biological and physical proxy data to reconstruct
temperatures far into the Earth's past. The accuracy
of these reconstructions is dependent on how closely
the climatic variable influences the phenomenon of
study. Error can creep into these analyses if some
other variable (besides the climatic variable) is also
influencing the proxy phenomenon.
The Earth's climate is variable. Over
the last billion years, global average temperature
has fluctuated by as much as 15 degrees Celsius from
the value observed today. For much of this history,
average global temperatures have been warmer. There
have, however, been periods when temperatures were
much colder and glaciers covered vast regions on the
Earth's surface. For example, for much of the past
2 million years global temperatures have been 2 to
5 degrees cooler than they are today. This climate
caused the formation of vast continental ice sheets
over most of North America and in selected regions
in Europe and Asia. The past 14,000 years have been
a time of glacial retreat because of generally warmer
global temperatures.
A variety of mechanisms can cause climate
change. Four of the most important mechanisms are examined
in detail. The first mechanism involves variations
in the Earth's orbital characteristics. The orbital
characteristics that are involved include changes in
the Earth's tilt, variations in the timing of aphelion
and perihelion, and changes to the shape of the Earth's
orbital path around the Sun. As the Earth travels through
space, these three separate cyclic variations combine
to produce long-term variations in the amount of solar
radiation received by the Earth's surface. The amount
of carbon dioxide in the Earth's atmosphere influences
the strength of the atmosphere's greenhouse effect.
Natural variations in the concentration of carbon dioxide
occur because of changes in global mean temperature.
When global temperatures are warm there is a net movement
of carbon dioxide from the oceans into the atmosphere.
Colder mean temperatures reverse this process, lowering
the concentration of carbon dioxide in the atmosphere.
Since the Industrial Revolution, humans have been artificially
increasing the concentration of carbon dioxide in the
atmosphere through the burning or fossil fuels and
the alteration of ecosystems. Volcanic eruptions can
cause short-term cooling of the Earth's climate. This
cooling occurs because some volcanic eruptions release
material into the stratosphere that effectively blocks
the reception of solar radiation by the Earth's surface.
Eruptions that can cause climatic change must be rich
in sulfur dioxide. The final mechanism that can cause
climate change is variations in the Sun's output of
radiation. Satellite measurements indicate that the
Sun's output can vary by as much as 0.1% over a 18
month period. A variation of 1% would cause average
global temperature to change by 1 degree Celsius.
El Niño is the name given to the infrequent
development of warm ocean surface waters along the
coast of Ecuador and Peru. It develops when there is
change in the circulation of the atmosphere across
the tropical pacific. Globally, the development of
El Niño is also associated with a number of other climatic
changes in other parts of the world.
List of Key Terms
Adiabatic, Absorption, Advection, Air
Mass, Albedo, Altocumulus
Clouds, Altostratus
Clouds, Ammonia, Angle
of Incidence, Anticyclone, Aphelion, Atmospheric
Pressure, Autumnal
Equinox,
Blizzard,
Carbon
Dioxide, Centripetal
Acceleration, Centripetal
Force, CFC, Chlorofluorocarbons, Cirrus
Clouds, Cirrostratus
Clouds, CLIMAP
Project, Climatic
Optimum, Cold
Front, Condensation, Condensation
Nuclei, Conduction, Convection, Convergence, Coriolis Force, Counter-Radiation, Cumulus, Cumulonimbus, Cumulus
Clouds, Cyclogenesis, Cyclone, Cyclonic,
Day
Length, Denitrification, Density, Deposition, Dew
Point, Diffused Solar
Radiation, Diffused
Insolation, Diffusion, Disturbance, Direct
Insolation, Direct
Solar Radiation, Divergence, Dry
Line,
Easterly
Wave, Eccentricity, El
Niño, Energy, Environmental
Lapse Rate, Equinox, Evaporation, Eye,
Ferrel
Cell, Force, Force
of Acceleration, Friction, Frictional
Force, Frictional
Deceleration, Front, Frontal
Cyclone, Frontal
Uplift, Frontal Zone,
Geostrophic Wind, Glacial, Gradient Wind, Gravity, Greenhouse
Effect, Greenhouse
Gas, Gust
Front,
Hadley
Cell, Hail, Heat, Heat
Energy, High
Pressure, Holocene
Epoch, Horizon, Hurricane,
Ice
Age, Ideal
Gas Law, Industrial
Revolution, Infrared
Radiation, Interglacial, Intertropical
Convergence Zone, Insolation, Isobar, Isoline, Isotherm, Isothermal,
Land
Breeze, Langley, La
Niña, Latent
Heat, Latent
Heat Flux, Lightning, Little
Climatic Optimum, Little
Ice Age, Longwave
Radiation, Low
Pressure,
Mass, Meridional, Meridional
Transport, Mesopause, Mesosphere, Methane, Mid-Latitude
Cyclone, Milankovitch Theory, Millibar, Monsoon, Montreal
Protocol, Mountain
Breeze,
Net
Radiation, Newton, Nimbostratus
Clouds, Nitrogen
Fixing, Nitrous
Oxide, Northeast
Trades,
Obliquity, Occluded Front, Ocean
Current, Orographic Lifting, Orographic
Uplift, Ozone, Ozone
Hole, Ozone
Layer,
Perihelion, Photochemical
Smog, Photosynthesis, Pleistocene, Polar
Axis, Polar
Cell, Polar
Front, Polar
High, Polar
Jet Stream, Polar
Stratospheric Cloud, Polar
Vortex, Potential
Evapotranspiration, Precession
of the Equinox, Precipitation, Pressure, Pressure
Gradient, Pressure
Gradient Force, Proxy
Data,
Radiometer, Rain, Reflection, Relative
Humidity, Respiration, Roll
Cloud,
Scattering, Sea
Breeze, Sensible
Heat, Sensible
Heat Flux, Shortwave
Radiation, Solar
Insolation, Solar
Radiation, Solstice, Source
Region, Southeast
Trades, Southern
Oscillation, Specific
Heat, Stationary
Front, Stratocumulus
Clouds, Stratosphere, Stratus
Clouds, Subpolar
Lows, Subtropical
High Pressure Zone, Subtropical
Jet Stream, Sulfur
Dioxide, Sun, Sunspots,Specific
Heat, Standard
Sea-Level Pressure,Solar
Noon, Solstice, Stratopause, Stratosphere, Summer
Solstice, Sun, Surface
Heat Flux,
Temperature, Terminal
Velocity, Thermal
Circulation, Thermal
High, Thermal
Low, Thermal
Wind System, Thermosphere, Thunder, Thunderstorm, Tornado, Total
Column Ozone, Trade
Winds, Transpiration, Troposphere, Tropopause, Trough,
Ultraviolet
Radiation, Urban
Heat Island,
Valley
Breeze, Venturi, Vernal
Equinox, Volcanic, Volume,
Warm
Front, Waterspout, Watt, Wein's
Law, Westerlies, Wind,
Y-axis, Younger-Dryas,
Zonal
Study Questions,
Problems, and Exercises
Essay Questions
(1). Describe and
compare the daily cycles of insolation, net radiation
and temperature for Kelowna (50 degrees North latitude)
on an average June 21st and December 22nd. In your
essay, be sure to include information on the height
of the Sun at solar noon (A=90-L±23.5),
length of daylight for each of these days (approximately
8 vs 16 hrs), and the timing of minimum and maximum
daily temperatures.
(2). How does the tilt of the Earth's
axis influence the annual solar insolation received
at a site located at 50 degrees South latitude?
(3). Why is ozone important for life
on Earth? Where is it found and how is it formed?
How is human activity influencing this important
atmospheric gas?
(4). How is the incoming shortwave
solar radiation from the Sun modified by the atmosphere
and the Earth's surface?
(5). Describe the difference between
the following two terms: heat and temperature.
(6). Describe the shortwave radiation
cascade as it relates to the Earth's energy balance
(see the following Link)?
(7). Discuss how the Greenhouse Effect
works? How has human activity over the last few centuries
enhanced this natural process? How will global warming
change the environment of the Earth?
(8). Discuss the following energy balance
equation in terms of two locations: the beautiful
island of Hawaii and the highland plateau of Mongolia
in the winter.
Q*
= Kdown - Kup + Ldown - Lup
where:
Kdown is
income direct and diffuse solar radiation or insolation.
Kup is
shortwave radiation reflected from the Earth's
surface back to space.
Ldown is
counter-radiation because of the greenhouse effect.
Lup is
the emission of longwave radiation from the Earth's
surface back to space.
(9). Name and describe the characteristics
of the various layers found in the atmosphere.
(10). What is a hurricane? Where, when
and why does it form? How is global warming going
to influence hurricane intensity and frequency.
(11). In a diagram, indicate the fronts,
wind directions, pressure pattern, and precipitation
pattern associated with a mature mid-latitude cyclone.
(12). The following diagram describes
the major pressure systems on the surface of the
Earth. On this diagram sketch in the surface wind
directions associated with these pressure systems
on an Earth which is spinning clockwise from the
northpole.
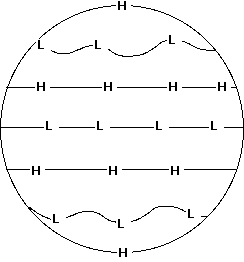
(13). Discuss the formation and characteristics
of the various types of thunderstorms.
(14). Describe the climatic characteristics
of the following Koppen Climate Classifications:
Cfa, Cs, and Cfb.
(15). What factors are responsible
for the altered micro-climate of urban areas?
(16).
Why does the jet stream in the
Northern Hemisphere move north in the summer and
south in the winter ?
(17). Four mechanisms of climatic change
were discussed in class. What were they, how do they
function and what type of time scale do they operate
in.
(18). When did the Little Ice Age occur
and how much colder was the global temperature at
this time?
(19). How can we reconstruct past ocean
temperature from the plankton species known as Foraminifera?
(20). Outline the climatic characteristics
and associated weather phenomena of the following
three Koppen climate regimes Am, Af, and Aw.
(21). Why do urban areas have more
energy available for the creation of sensible heat
than rural areas?